A Race Against Time: How Oligonucleotides Are Changing Lives
When Sarah was born, everything seemed normal. But her parents noticed something unusual as she grew—her growth was markedly different from the other kids. At two years of age, she struggled to walk. By three, she could barely hold a spoon. After running a series of tests, doctors concluded that Sarah had a rare genetic disorder.
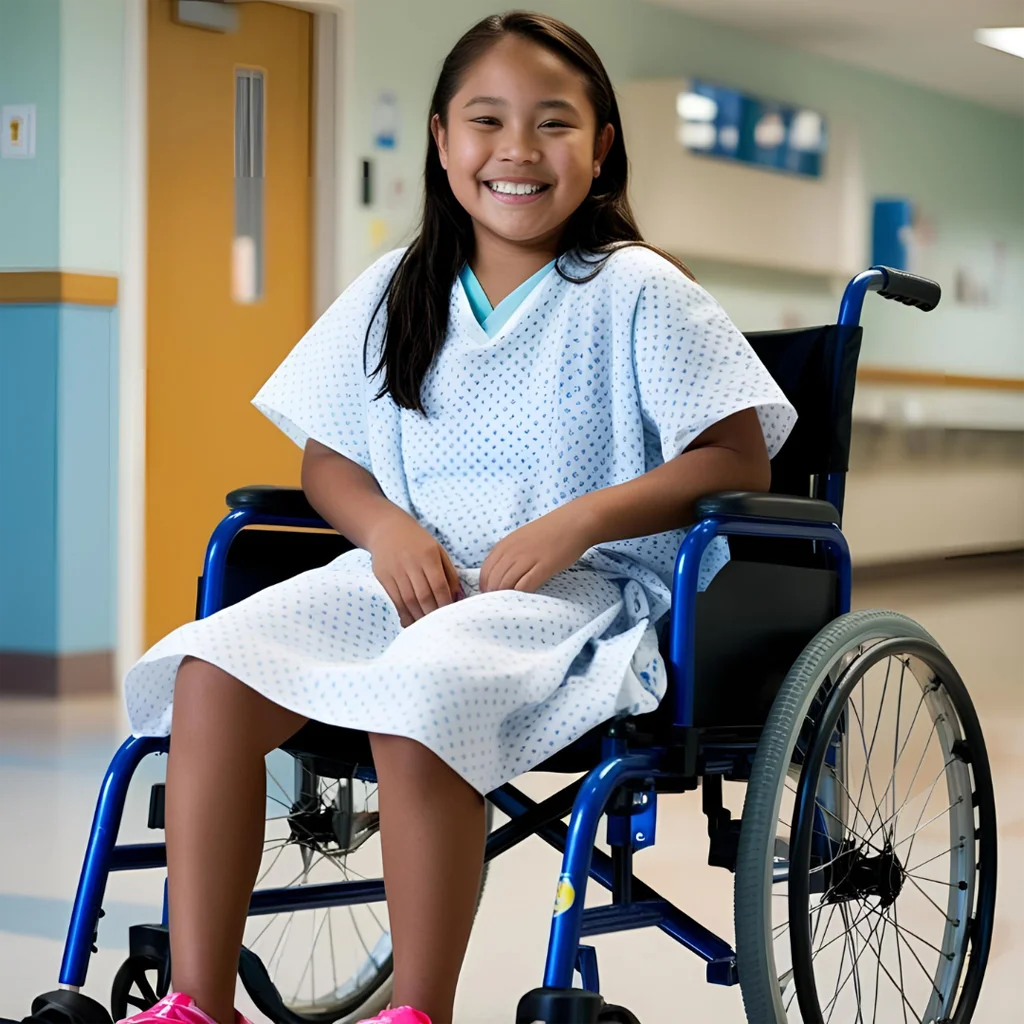
A genetic disorder occurs when a small error in an individual’s DNA disrupts how their body functions. Some of these errors are inherited, whereas others occur by chance. They can cause serious conditions such as muscle diseases, neurological disorders, and even life-threatening illnesses. For Sarah, the faulty gene meant her body could not produce a critical protein needed for muscle movement. Unfortunately, there was no cure for Sarah’s disease.
Her parents were devastated. But then, a team of scientists and doctors suggested a cutting-edge approach—oligonucleotide therapy.
What is Oligonucleotide Synthesis?
In living organisms, DNA and RNA are naturally produced through replication and transcription processes, respectively. However, as the demand for specific DNA and RNA sequences has increased, owing to genetic research, diagnostics, and therapeutics, laboratory-based methods have been developed to synthesize oligonucleotides in large quantities. Therefore, oligonucleotide synthesis is the process of building short strands of DNA or RNA in a laboratory. These custom-made sequences are designed to precisely target and correct faulty genetic instructions, such as a biological "edit" that fixes the root cause of a disease. The first chemical synthesis of DNA was achieved in the 1950s, with major advancements occurring in the 1970s when phosphoramidite chemistry was introduced, making oligonucleotide synthesis more efficient and scalable.
DNA sequences synthesized in the lab are often referred to as oligonucleotides because they are short-chain nucleic acids, typically composed of 10 to 100 nucleotides. The term "oligonucleotide" originates from the Greek words oligo (meaning few or small) and nucleotide (the fundamental building block of DNA and RNA). These synthetic sequences play a crucial role in research, enabling applications such as PCR (Polymerase Chain Reaction), gene editing, antisense therapy, and CRISPR-based genome modifications.
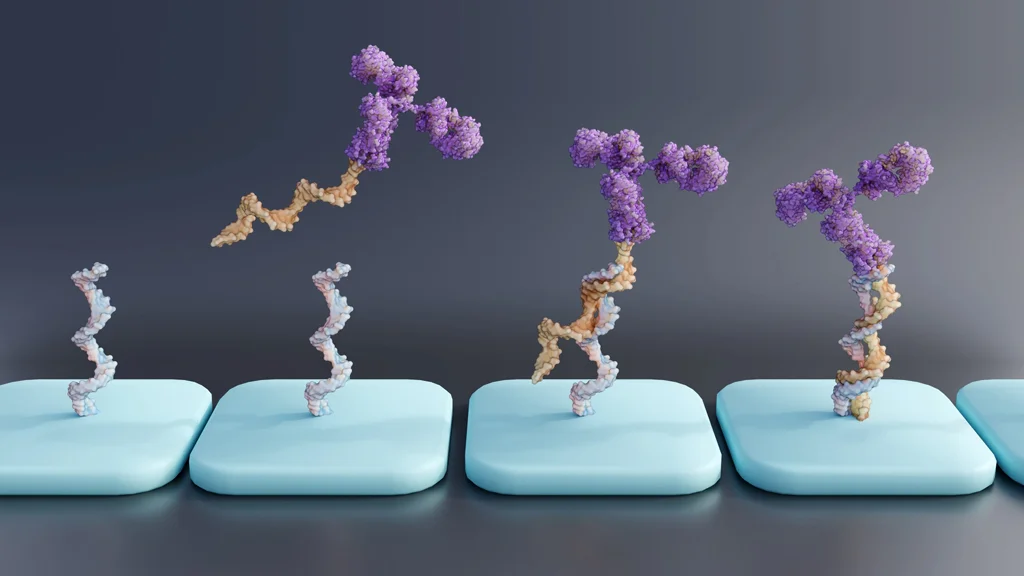
With the rapidly growing importance of gene-based therapies and molecular diagnostics, oligonucleotide synthesis has evolved into an essential technology for advancing medicine, enabling scientists to design and produce tailored genetic sequences with high precision.
For Sarah, the oligonucleotide therapy implied designing an oligonucleotide that could silence the defective gene and allow her body to work properly. Scientists carefully engineered the molecule, ensuring it was pure, stable, and effective. After rigorous testing, Sarah received her first dose.
How it worked
Once inside her body, the oligonucleotide acted like a tiny messenger, binding to the faulty genetic code and preventing it from making harmful proteins. Over time, the treatment helped her regain some muscle function. She began to move more easily, hold her parents' hands, and even take her first steps. Her parents never thought this would be ever possible!
Sarah’s story is just one of many. Oligonucleotides are revolutionizing medicine, offering hope for patients with rare diseases, cancers, and even viral infections. But developing these molecules at scale—ensuring their precision, purity, and regulatory compliance—is challenging.
At Aurigene Pharmaceuticals, we specialize in high-quality oligonucleotide synthesis, supporting pharma and biotech companies in bringing life-changing therapies to the world. Our expertise, cutting-edge facilities, and commitment to excellence ensure that when science pushes the boundaries of what’s possible, we are there to make it a reality.
Connect with our scientific experts for your drug discovery, development and manufacturing needs
Oligonucleotides and Their Synthesis: A Cornerstone of Modern Molecular Science
Structure and function of oligonucleotides
Oligonucleotides are composed of a sequence of nucleotides, the fundamental units of nucleic acids. Each nucleotide consists of three key components: a nitrogenous base, a pentose sugar, and a phosphate group. The nitrogenous base determines the genetic code, with adenine (A), thymine (T), cytosine (C), and guanine (G) found in DNA, whereas uracil (U) replaces thymine in RNA. The pentose sugar provides structural support, with deoxyribose present in DNA and ribose in RNA. The phosphate group plays a crucial role in linking nucleotides together, forming the backbone of the oligonucleotide strand. This structured arrangement allows oligonucleotides to function in various biological and synthetic applications, such as gene regulation, diagnostics, and therapeutics.
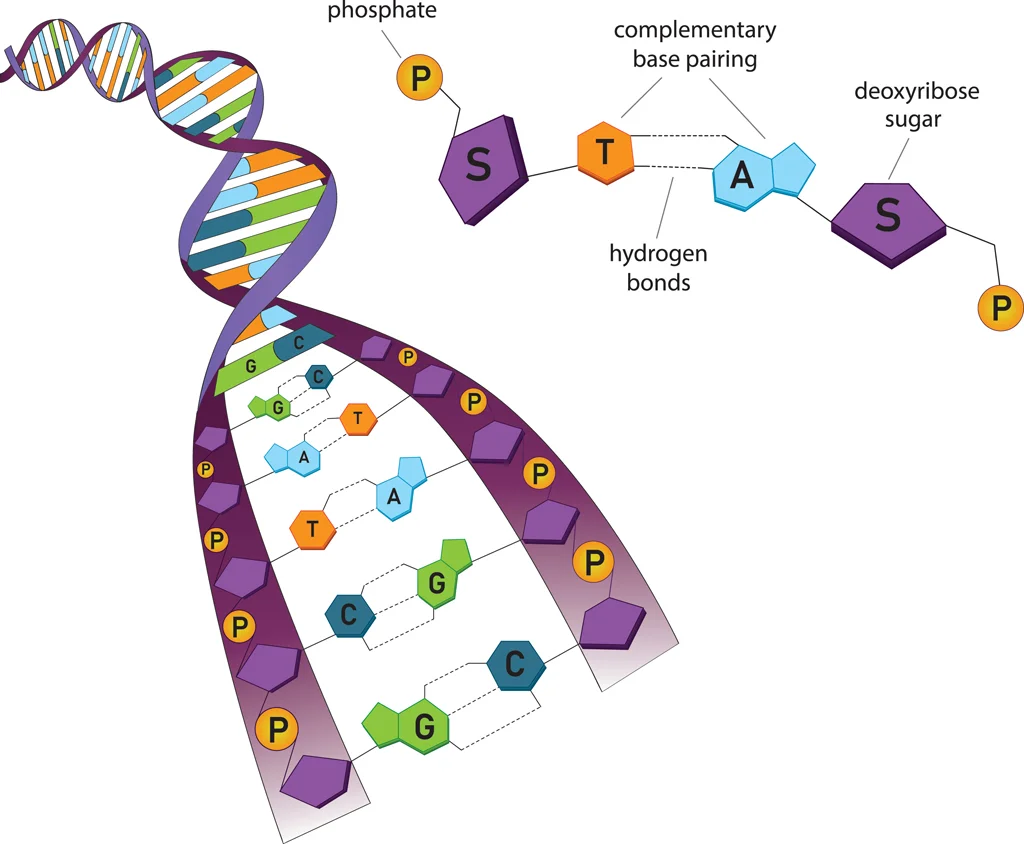
Oligonucleotides function by hybridizing, or binding, to complementary sequences of nucleic acids through base pairing, enabling their use in various applications. In PCR, oligonucleotides act as primers that initiate DNA amplification, making them essential for genetic analysis and research. In gene silencing, antisense oligonucleotides (ASOs) and small interfering RNAs (siRNAs) regulate gene expression by binding to target mRNA sequences and preventing protein synthesis. Diagnostic tests leverage oligonucleotides to detect genetic mutations, pathogens, or disease biomarkers, aiding in early disease detection and precision medicine. In therapeutics, oligonucleotide-based drugs are used to treat conditions like spinal muscular atrophy (SMA) and Duchenne muscular dystrophy by targeting faulty genetic sequences and correcting gene expression. Through these diverse applications, oligonucleotides play a crucial role in advancing molecular biology, diagnostics, and precision medicine.
Solid-Phase Oligonucleotide Synthesis: The Standard Method
The most widely used technique for oligonucleotide synthesis is solid-phase synthesis, a stepwise chemical process that enables the sequential addition of nucleotides to a growing chain while attached to an insoluble support, such as controlled porous glass or polymer beads. This method ensures high purity, efficiency, and scalability while preventing unwanted side reactions. The automation of solid-phase synthesis enables the precise control of sequence composition, ensuring that oligonucleotides meet high standards of accuracy, stability, and yield.
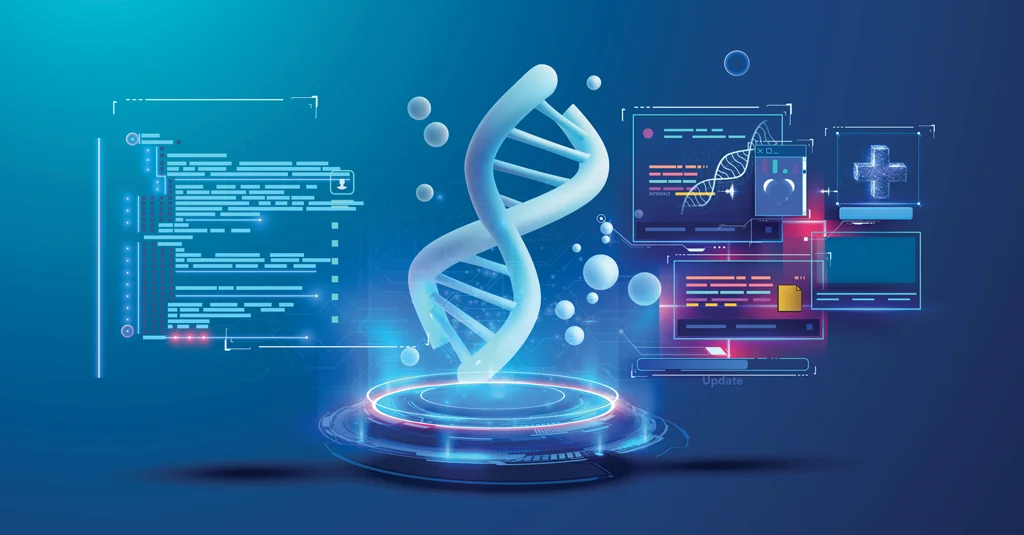
The synthesis process involves several key steps. It begins with deblocking, where a dimethoxytrityl (DMT) group is removed from a protected nucleoside attached to the solid support. This exposes the 5' hydroxyl (-OH) group, making it ready for the addition of the next nucleotide. The coupling step follows, where the next nucleotide—attached to a phosphoramidite group—is chemically activated and added to the growing oligonucleotide strand. A catalyst ensures that efficient bonding occurs, minimizing errors in synthesis.
To maintain sequence integrity, any unreacted sites undergo capping, a process that blocks incomplete sequences from further extension, ensuring the final product remains highly pure. Next, the newly formed phosphate backbone undergoes oxidation, stabilizing the phosphite linkage into a more durable phosphate form. These steps—deblocking, coupling, capping, and oxidation—are repeated until the full-length oligonucleotide is synthesized with the desired sequence.
Once synthesis is complete, the oligonucleotide undergoes cleavage and deprotection, where it is detached from the solid support, and protective chemical groups are removed, revealing the functional nucleic acid strand. Finally, the synthesized oligonucleotide undergoes purification and quality control to eliminate incomplete sequences and ensure accuracy. Techniques like high-performance liquid chromatography (HPLC) or gel electrophoresis help in purification, while mass spectrometry and capillary electrophoresis confirm the oligonucleotide’s sequence integrity and chemical purity.
This automated and highly optimized process makes solid-phase synthesis the gold standard for large-scale oligonucleotide production, facilitating the development of primers, probes, gene-silencing tools, and therapeutic molecules with high fidelity and reproducibility. With ongoing advancements in synthesis chemistry and purification techniques, the efficiency, cost-effectiveness, and scalability of oligonucleotide manufacturing continue to improve, paving the way for broader applications in genomics, molecular diagnostics, and personalized medicine.
Applications of Synthetic Oligonucleotides
The ability to design and synthesize oligonucleotides with high precision has revolutionized multiple fields of science and medicine. These short nucleotide sequences serve as essential tools in molecular diagnostics, genetic research, therapeutics, and genome editing. Their adaptability allows them to be customized for specific targets, making them invaluable in applications ranging from disease detection to advanced genetic engineering.
One of the most critical applications of synthetic oligonucleotides is in molecular diagnostics. They are widely used as probes to detect genetic mutations, viral RNA, and bacterial DNA, aiding in the early and accurate diagnosis of genetic disorders and infectious diseases. A notable example is their role in COVID-19 testing, where oligonucleotide-based probes help identify the presence of SARS-CoV-2 RNA through PCR-based techniques. Their high specificity enables reliable pathogen detection, facilitating timely medical interventions.
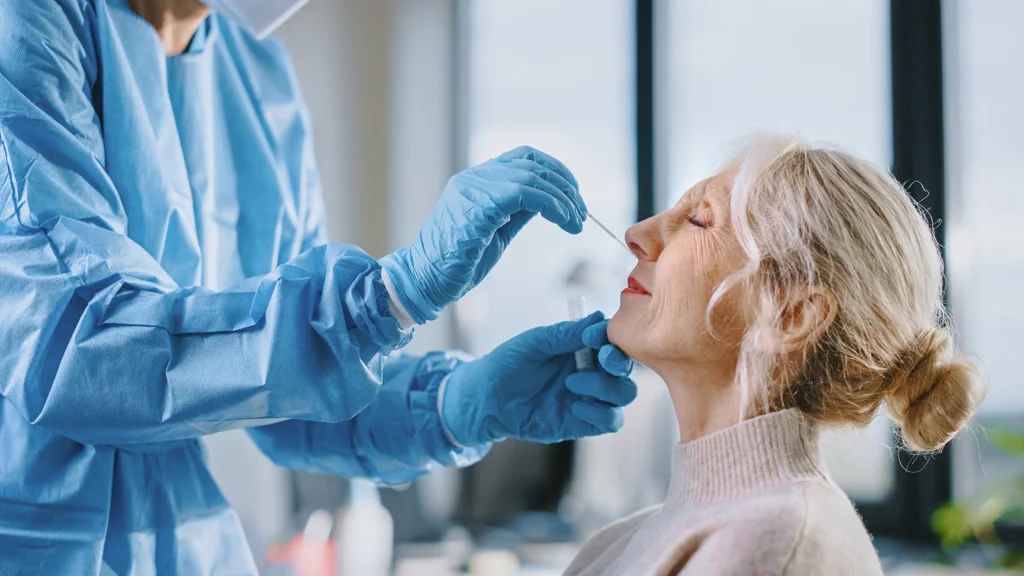
In polymerase chain reaction (PCR) and quantitative PCR (qPCR), oligonucleotide primers play an essential role in DNA amplification. These primers bind to specific DNA sequences and initiate replication, enabling genetic analysis for forensic investigations, clinical diagnostics, and molecular biology research. The widespread use of PCR in genetic fingerprinting, paternity testing, and pathogen detection highlights the indispensable role of synthetic oligonucleotides in modern science.
Another significant application is in gene silencing and RNA interference (RNAi). ASOs and siRNAs regulate gene expression by binding to complementary mRNA sequences, preventing translation into proteins. This mechanism is particularly useful in treating genetic disorders where overactive or faulty genes need to be silenced to restore normal cellular functions. RNAi-based therapeutics are being explored for treating neurodegenerative diseases, viral infections, and certain cancers.
Synthetic oligonucleotides are also making a profound impact in therapeutic applications. Several FDA-approved oligonucleotide-based drugs have transformed the treatment of previously untreatable conditions. For example, Spinraza (nusinersen), an antisense oligonucleotide therapy, has provided life-changing benefits for patients with spinal muscular atrophy (SMA) by modifying gene splicing to restore protein function. Similarly, in Duchenne muscular dystrophy (DMD), exon-skipping therapies such as eteplirsen (Exondys 51) help the body produce partially functional dystrophin proteins, improving mobility and slowing disease progression. Oligonucleotide-based drugs are also being developed for cancer treatments, where they target oncogenes, enhance immune responses, and block the expression of tumor-promoting genes.
In the field of CRISPR and genome editing, synthetic oligonucleotides play a crucial role in guiding gene modifications. Synthetic guide RNAs (gRNAs) direct the CRISPR-Cas9 enzyme to specific genomic locations, enabling precise gene editing. This technology has revolutionized genetic engineering, offering potential cures for genetic disorders, improved agricultural traits, and novel approaches to disease modeling. The specificity and efficiency of CRISPR, facilitated by synthetic oligonucleotides, are paving the way for advancements in precision medicine.
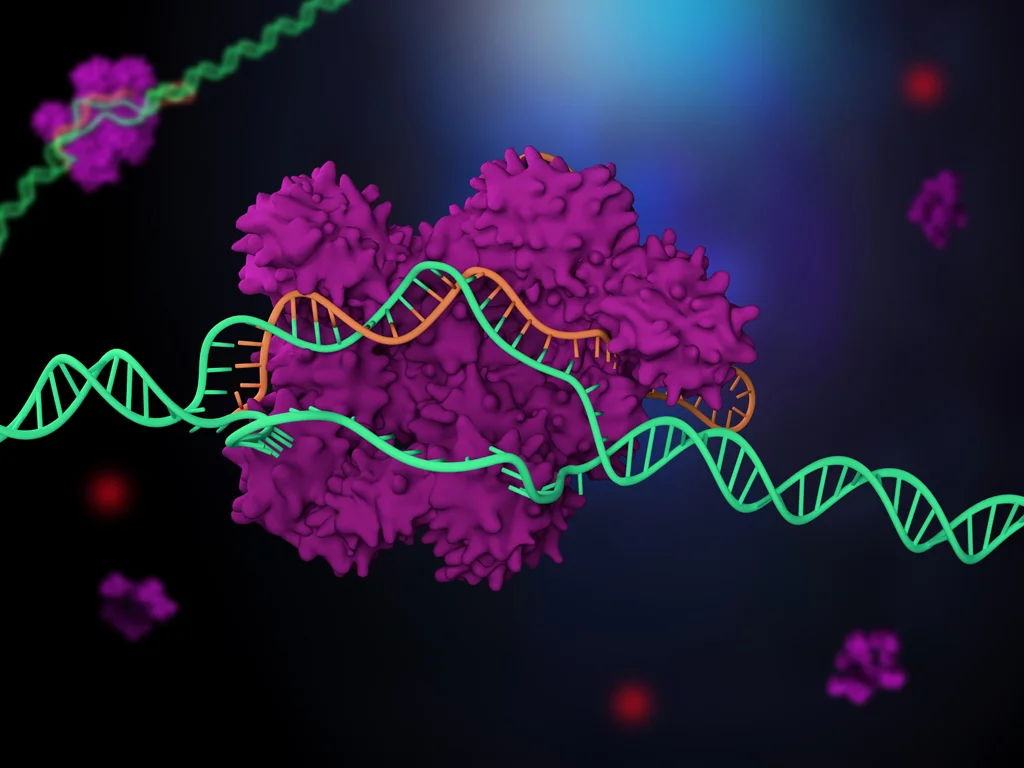
Another innovative application of oligonucleotides is in the development of aptamers, which serve as synthetic antibody alternatives. Unlike traditional antibodies, aptamers are short DNA or RNA sequences that fold into three-dimensional structures and bind selectively to target proteins or small molecules. They are used as molecular recognition elements in drug development, diagnostics, and targeted therapies. Aptamers offer advantages such as high stability, reduced immunogenicity, and the ability to be chemically synthesized with high reproducibility. These properties make them ideal for therapeutic and diagnostic applications, including targeted drug delivery and biomarker detection in diseases like cancer and cardiovascular conditions.
The broad spectrum of applications for synthetic oligonucleotides continues to expand as research advances. With ongoing improvements in synthesis techniques, delivery mechanisms, and regulatory approvals, oligonucleotide-based technologies are set to play an even greater role in the future of medicine, diagnostics, and biotechnology.
General Services Offered by CDMOs in Oligonucleotides
CDMOs play a crucial role in advancing oligonucleotide-based therapeutics, offering a comprehensive suite of services that facilitate the transition from early-stage research to commercial production. These services include:
1. Custom Oligonucleotide Synthesis
Tailored synthesis of oligonucleotides is available at various scales, accommodating everything from small quantities for research to larger batches for clinical trials and commercial use. Advanced synthesis services also include characterization and biological evaluation, supported by state-of-the-art facilities and skilled scientists.
2. Process Development and Optimization
Developing robust and efficient manufacturing processes is crucial for producing high-quality oligonucleotides. Expertise in process optimization enhances yield, purity, and scalability, supporting early-stage development through Phase II/III clinical supply, with commercial supply expected in the coming years.
3. Analytical Method Development and Validation
Accurate analytical methods are essential for characterizing oligonucleotides and ensuring they meet stringent quality standards. Comprehensive analytical development services are available to facilitate regulatory compliance and product consistency.
4. Scale-Up and cGMP Manufacturing
Transitioning from laboratory-scale synthesis to large-scale manufacturing requires expertise in scaling up processes while adhering to current Good Manufacturing Practices (cGMP). Process development, scale-up, and cGMP manufacturing at various scales support early-stage development through to clinical and commercial supply.
5. Drug Product Formulation and Fill-Finish Services
Beyond synthesizing the active pharmaceutical ingredient (API), formulation development ensures the oligonucleotide’s stability, bioavailability, and delivery to the target site. Comprehensive drug product support services include bioavailability enhancement and lipid nanoparticle (LNP) formulation and manufacturing. Fill-finish services prepare the final drug product in its delivery form, such as vials or syringes.
6. Regulatory Support and Quality Assurance
Navigating the regulatory landscape is complex, requiring expert support in preparing documentation, ensuring compliance with international standards, and implementing rigorous quality assurance protocols. These services ensure that oligonucleotide therapeutics meet all necessary regulatory requirements for clinical and commercial use.
Market Growth and Opportunities
The oligonucleotide CDMO market is experiencing significant growth, driven by the increasing focus on developing and commercializing oligo-based therapeutics and the outsourcing of development and manufacturing processes. The market was valued at approximately $2.33 billion in 2023 and is projected to reach $6.73 billion by 2029, reflecting a compound annual growth rate (CAGR) of 21.8%.
By offering these integrated services, pharmaceutical and biotechnology companies can efficiently advance oligonucleotide-based therapies from concept to market, ensuring quality, scalability, and compliance at every stage.
What Aurigene offers
- State-of-the-Art Laboratory: 1000 sq. ft dedicated lab space with humidity control.
- Synthesis Equipment:
- K & A H-16 and 8 automated DNA/RNA synthesizer
- Shimadzu preparative RP-HPLC
- AKTA pure 25M FPLC Ion-exchange purification system
- Sartoflow Smart TFF system for concentration and desalting
- Martin Christ LSC Plus Lyophilizer with lyocube, benchtop tray lyophilizer
- Analytical Instruments:
- edicated Agilent LC-MS for oligonucleotide analysis (up to 100K da)
- Implen N60 nano spectrophotometer
Aurigene services
Tailored oligonucleotide synthesis solutions
- Oligonucleotide Synthesis:
- Scale: 1-250 µmol (1-1000 mg)
- 16x1 µmol, 8x40 µmol, 1x250 µmol
- Size: 3 to 60-mer
- 2’-F, 2’-OMe, 2’-O-MOE, LNA and all standard modifications
- Post-synthetic conjugation of Lipids, Peptides, GalNAc, Antibodies, and Chelators at 3’/5’-end of desired oligo.
- Duplexing of antisense and sense strand with >95% purity
- Purification and Characterization:
- Ion exchange and reverse phase HPLC
- Size exclusion for duplex analysis and desalting
- UV quantification, Purity and identity analysis using LC-MS (TOF) and mass deconvolution
- HPLC with different mobile and stationary phase for purity assessment
- Biological Evaluation:
- In vitro IC50, Kd, and binding assessment
- In vivo efficacy studies in oncology and inflammation models
- Toxicology studies for ASOs
- Conjugation Capabilities:
- Acid-amine coupling using direct acid or through NHS chemistry
- Alkyne-azide coupling with/without copper
- S-S and C-S bond formation by disulfide exchange or thiol-ene click
- Expertise in Oligonucleotide Chemistry:
- Custom phosphoramidite synthesis for specialized modifications
- Proficiency in nucleoside, sugar, and base modifications
- Advanced Conjugation Techniques:
- Click chemistry, amide coupling, and thiol-mediated conjugation
- Integrated Drug Discovery Solutions:
- CADD (Computer-Aided Drug Design) optimization
- Comprehensive services from synthesis to biological evaluation
- End-to-End Oligonucleotide Development:
- Expertise in optimizing oligonucleotide design and assay iterations
- Seamless transition from synthesis to biological evaluation
Aurigene Pharmaceutical Services offers a fully integrated oligonucleotide platform, leveraging cutting-edge infrastructure, specialized expertise, and a robust service portfolio to support drug discovery and therapeutic development.
Challenges and Future Directions in Oligonucleotide Synthesis and Therapeutics Industry
The oligonucleotide synthesis and therapeutics industry is experiencing rapid growth, driven by advancements in genetic research and the development of targeted treatments for various diseases. Oligonucleotides have transitioned from experimental concepts to an established therapeutic class alongside small molecules and biologics. Continuous innovations in synthesis, delivery, and regulatory frameworks are poised to further transform the industry. However, challenges related to efficiency, cost, scalability, regulatory compliance, and the emerging demand for personalized medicine continue to impact the field. Addressing these hurdles is crucial for unlocking the full potential of oligonucleotide-based therapeutics and diagnostics.
Expanding Therapeutic Applications
Oligonucleotides have become essential tools in treating genetic disorders, viral infections, and cancers owing to their ability to precisely modulate gene expression. Over 13 oligonucleotide-based drugs have received regulatory approval, targeting diseases affecting the liver, central nervous system (CNS), skeletal muscle, and the eye, with future developments anticipated for applications in the lung, kidney, and bone marrow. ASOs and siRNAs silence specific genes by targeting messenger RNA (mRNA), reducing the production of disease-causing proteins. Emerging gene modulation techniques, such as epigenetic therapies, are also being explored to treat chronic infections like Hepatitis B by modifying viral gene expression without altering the host genome.
Despite these promising advancements, the production of high-purity oligonucleotides on a large scale remains expensive due to stringent requirements for chemical reagents, purification, and quality control. The phosphoramidite chemistry used in solid-phase synthesis is costly, and the need for ultra-pure reagents adds to the financial burden. Researchers are now exploring alternative synthesis approaches, such as enzymatic synthesis and click chemistry, to lower costs and improve efficiency while maintaining high fidelity in sequence synthesis.
Advances in Oligonucleotide Chemistry and Manufacturing
To enhance the stability and efficacy of oligonucleotide drugs, extensive chemical modifications have been explored. Phosphorothioate (PS) linkages improve nuclease resistance and pharmacokinetics, though their stereochemistry presents challenges. Advancements in stereopure synthesis methods allow for precise control of these linkages, improving potency while reducing toxicity. Modifications such as locked nucleic acids (LNAs) and 2’-O-methyl (2’-OMe) enhance binding affinity, increasing the effectiveness of oligonucleotide therapies.
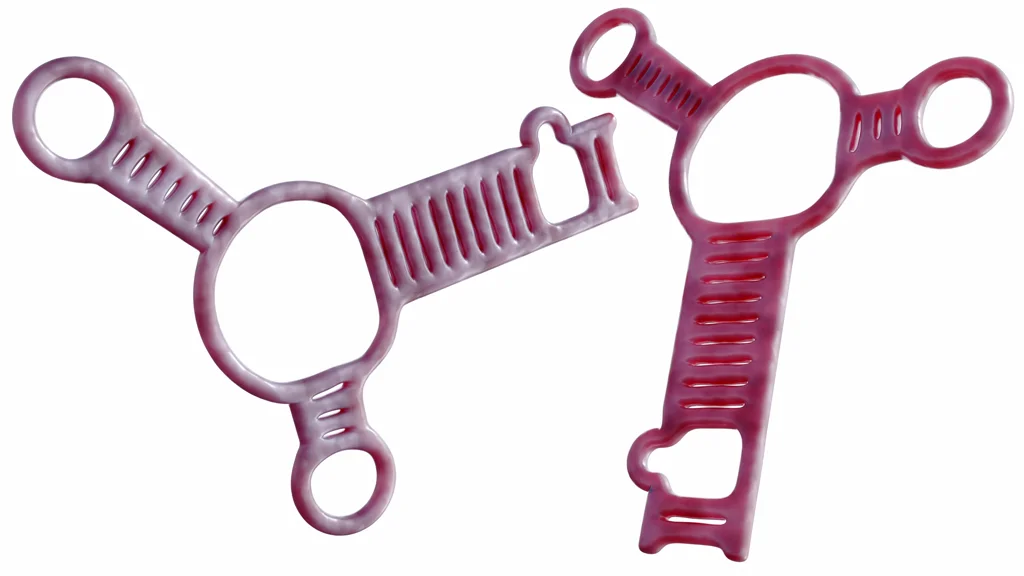
While modern synthesis techniques are highly automated, inefficiencies in the chemical coupling of nucleotides can lead to incomplete sequences, reducing the final yield of full-length oligonucleotides. Each step in the synthesis process has a small probability of failure, and these errors accumulate, necessitating extensive purification steps such as high-performance liquid chromatography (HPLC) or ion-exchange chromatography. However, these purification methods increase complexity, add costs, and may lead to material loss. Optimizing coupling efficiencies and developing more robust purification techniques remain key areas of focus for the industry.
Innovative synthesis and manufacturing approaches, including enzymatic synthesis and Multicolumn Countercurrent Solvent Gradient Purification (MCSGP), provide sustainable alternatives to traditional chemical synthesis, reducing solvent waste and improving yield. Reducing the cost of oligonucleotide synthesis is essential for making these molecules more accessible for both research and clinical applications.
Integration of Artificial Intelligence in Drug Design
Artificial intelligence (AI) is revolutionizing oligonucleotide drug design by optimizing sequences for efficacy and reducing off-target effects. AI models can predict oligonucleotide behavior, accelerating the drug discovery timeline by rapidly identifying potential therapeutic candidates. These advancements in AI-driven oligonucleotide design and high-throughput screening are enabling the development of highly specific and effective therapies, particularly for personalized medicine applications.
Stability and Delivery Challenges
Oligonucleotides, particularly those used for therapeutic applications, are inherently unstable in biological environments. Unmodified DNA and RNA sequences are rapidly degraded by nucleases, limiting their effectiveness as drugs since they must remain intact long enough to reach target cells. To address this, chemical modifications such as 2’-O-methyl modifications, phosphorothioate backbones, and locked nucleic acids (LNAs) are introduced to enhance stability and resistance to enzymatic degradation. Additionally, efficient delivery systems, such as lipid nanoparticles (LNPs) and conjugation with cell-penetrating peptides, are being developed to improve cellular uptake and tissue targeting. These advancements are helping to enhance the therapeutic viability of oligonucleotide-based drugs.
Stereochemistry in Oligonucleotide Therapeutics
The stereochemistry of PS linkages significantly influences the pharmacological properties of oligonucleotides. Defined stereopure modifications improve therapeutic efficacy by enhancing stability and reducing immunogenicity. A specific modification within PS gapmers, known as the trivalent stereo-motif (3'-SₚSₚRₚ-5'), provides both nuclease stability and RNase H compatibility, improving overall drug performance.
Regulatory Landscape and Standardization
The regulatory framework for oligonucleotide therapeutics remains complex because of their unique molecular properties. Oligonucleotide-based drugs require rigorous validation to ensure safety, efficacy, and consistency before clinical use. Regulatory bodies such as the U.S. Food and Drug Administration (FDA) and the European Medicines Agency (EMA) have established stringent guidelines for oligonucleotide therapeutics, including Good Manufacturing Practice (GMP) requirements. Meeting these regulatory standards involves extensive analytical testing, stability studies, and process validation, adding complexity to drug development pipelines.
The FDA has provided specific guidance addressing clinical pharmacology considerations for oligonucleotide therapeutic development programs, including characterizing the potential for QT interval prolongation, performing immunogenicity risk assessments, and assessing drug-drug interactions. As the field matures, manufacturers are working on standardizing GMP-compliant synthesis methods to streamline regulatory approval processes and accelerate the delivery of oligonucleotide-based treatments to patients.
Market Growth and Opportunities
The oligonucleotide Contract Development and Manufacturing Organization (CDMO) market is expanding rapidly, fueled by the growing demand for targeted genetic therapies. The industry was valued at approximately $2.33 billion in 2023 and is projected to reach $6.73 billion by 2029, with a Compound Annual Growth Rate (CAGR) of 21.8%. Companies are investing heavily in expanding their production capacity to meet this rising demand.
Addressing Rare Genetic Diseases and Personalized Medicine
Oligonucleotide therapies offer hope for treating rare genetic diseases through customized, sequence-specific interventions. Personalized medicine approaches, including the development of modular oligonucleotide platforms, enable rapid adaptation to different genetic disorders. Improved conjugation methods enable more precise drug delivery, enhancing treatment efficacy while minimizing side effects.
However, bringing these customized treatments to market presents unique challenges, including small-scale manufacturing constraints, regulatory hurdles, and high costs. As AI and automation improve, the feasibility of personalized oligonucleotide therapies is expected to grow, paving the way for precision medicine approaches that target genetic diseases at their root cause.
The Road Ahead
Despite these challenges, ongoing innovations in synthesis technology, delivery methods, and regulatory frameworks are driving the field forward. The future of oligonucleotide synthesis and therapeutics is promising, with advancements in chemical modifications, AI-driven design, sustainable manufacturing, and regulatory harmonization playing a critical role in shaping the industry. As researchers and pharmaceutical companies continue to refine the processes involved, oligonucleotide-based therapeutics are poised to become a cornerstone of precision medicine, offering new hope for patients with previously untreatable conditions.